Introduction
Anthropogenic carbon dioxide emissions have led to rising concerns of global warming. Carbon dioxide in the atmosphere is more efficient at trapping heat than the other components in the air, chiefly nitrogen (78%), oxygen (20.9%), and argon (0.9%). Carbon dioxide concentration in the atmosphere has risen from 280 ppm in the early 1900s to over 400 ppm today and continues to rise at a rate of several ppm per year. These rising concentrations have led to concerns in many nations regarding the impacts over the next century. As a result, new adsorbent materials are being investigated to capture carbon dioxide directly from the air.
In 2019, greenhouse gas emissions accounted for release of 6,558 million metric tons of carbon dioxide (equivalent) with the three largest sectors being transportation (29%), electricity generation (25%), and manufacturing (23%). (epa.gov) The transportation industry is the largest contributor to greenhouse gas emissions, and it is difficult to directly capture their emissions. Direct air capture (DAC) is an emerging technology capable of capturing carbon dioxide directly from the air and is feasible in nearly any location.
In this app note, we investigated direct air capture in silica alumina and zeolite 13X in both humid (40% relative humidity) and dry conditions. Additionally, polyethyleneimine (PEI) and tetraethylenepentamine (TEPA) were loaded into the structures of silica alumina and zeolite 13X to enhance their carbon dioxide adsorption affinity.
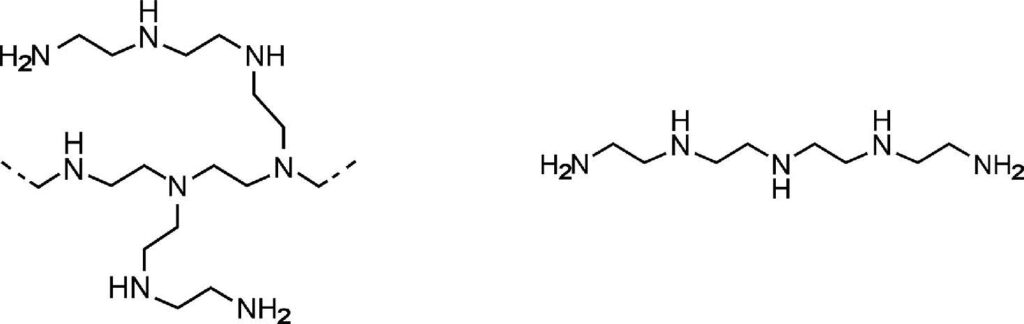
Experimental
Silica alumina reference material was obtained from Micromeritics and zeolite 13X was obtained from Zeochem. Materials were loaded with PEI by dissolving 4 mL of the amine in 20 mL of ethanol. Materials were loaded with TEPA by dissolving 10 mL of the amine in 20 mL of ethanol. The alumina or zeolite were then added (roughly 1.0g) and the mixture was stirred while heating to 50°C overnight. The solution was then disposed of, and the sample were washed with ethanol before being left out to dry.
Samples were activated for nitrogen physisorption measurements by activating under vacuum at 80°C overnight. Samples were analyzed using the standard conditions for mesoporous materials for SiAl and the standard conditions for microporous materials for zeolite 13X. Low temperature activation was used due to thermal decomposition of PEI or TEPA.
Samples were activated in-situ for breakthrough measurements under nitrogen flow while heating to 80°C for 12 hours. Samples were analyzed for breakthrough in dry conditions by mixing a stream of 800 ppm CO2 in nitrogen (10 sccm) with a pure nitrogen (10 sccm) stream. Helium (1sccm) was used as a tracer gas to determine the start of the breakthrough measurement.
Samples were analyzed for breakthrough in humid conditions by first saturating the sample with water, then collecting the humid CO2 breakthrough measurement. This method was used, as water and CO2 compete for adsorption sites and in “real world” process conditions, it is likely that the adsorbent would contain significant water loading during CO2 adsorption. Samples were first saturated with water by mixing a stream of wet nitrogen (8 sccm) with a stream of dry nitrogen (12 sccm). Next, humid CO2 breakthrough measurements were collected by mixing a stream of 800 ppm CO2 in nitrogen (10 sccm) with a dry nitrogen (1sccm) stream and a humid nitrogen (8 sccm) stream. Helium (1 sccm) was once again used as a tracer gas.
Results
Nitrogen Physisorption Analysis
Samples were analyzed for nitrogen physisorption analysis to determine their surface area. Samples were analyzed as-is as well as after loading with PEI and TEPA. We expected to see a drop in surface area after loading with PEI and TEPA as the amines would partially fill the pore space of the materials. Table 1 below shows the surface area results, and Figure 1 shows the collected isotherms for SiAl (left) and zeolite 13X (right).
The activation conditions for SiAl and zeolite 13X are typically 400 °C, this is not possible with PEI and TEPA loaded materials. Therefore, all materials were activated under the same conditions to ensure that PEI and TEPA were not removed from the samples during activation. Plain SiAl showed an acceptable surface area relative to what would be achieved under normal activation conditions (SA = 208 – 220 m2/g).
MATERIAL | Surface Area (m2/g) |
---|---|
SiAl Plain | 206.4 |
SiAl PEl | 95.6 |
SiAl TEPA | 131.6 |
Zeolite 13X Plain | 611.2 |
Zeolite 13X PEl | 2.9 |
Zeolite 13X TEPA | 4.9 |
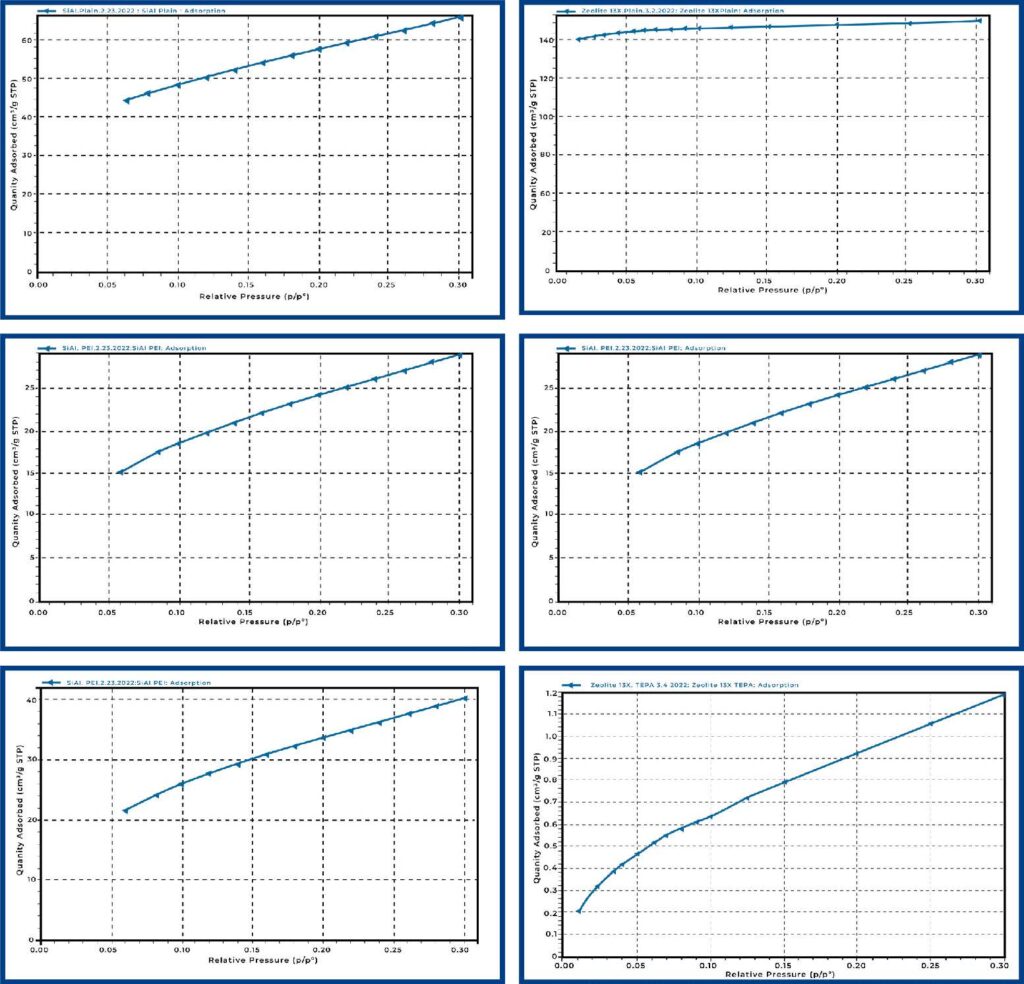
Breakthrough Measurements for Direct Air Capture
Carbon dioxide breakthrough measurements were collected on all materials in both humid and dry conditions. While the quantity adsorbed is low for all measurements, the time to breakthrough tended to be long due to the low concentration of CO2 (400 ppm). During all analyses, the He tracer gas breaks through completely shortly after the blending valves open, this occurs at 20 minutes (minimal dead time) for dry samples and after saturation with water for humid samples. Samples were first saturated with water prior to CO2 breakthrough measurements to better assess the competitive adsorption of CO2 with water. Table 2 in the conclusion section shows the quantity of CO2 adsorbed for all materials.
Plain Si-Al reference material was analyzed for direct air capture in both humid and dry conditions. The breakthrough curves are displayed in Figure 2 below. CO2 breaks through more gradually than the He tracer gas, which is expected as the concentration is very low, and the Si-Al pellets are large such that some mass transfer limitations are expected. Additionally, we observed a significant drop in capacity when comparing the dry and humid results, which is expected as CO2 and water compete for adsorption sites.
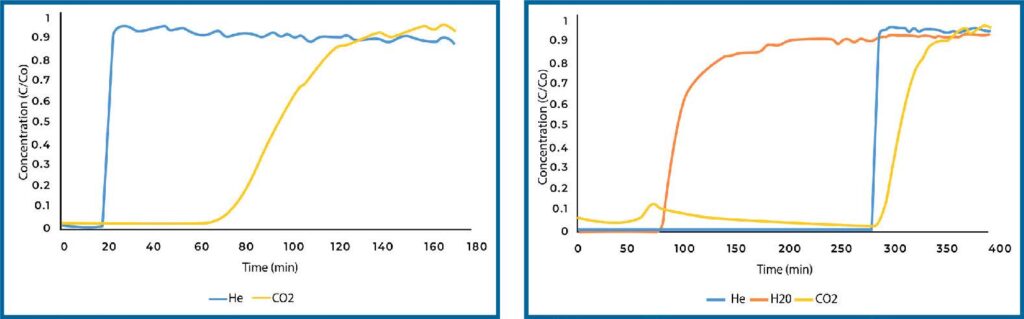
PEI loaded Si-Al displayed a significant increase in CO2 adsorption in dry conditions over plain Si-Al (see Figure 3). The quantity adsorbed increased by nearly four times. When analyzed under humid conditions, the quantity of CO2 adsorbed dropped significantly, however it still adsorbed more CO2 than plain Si-Al.
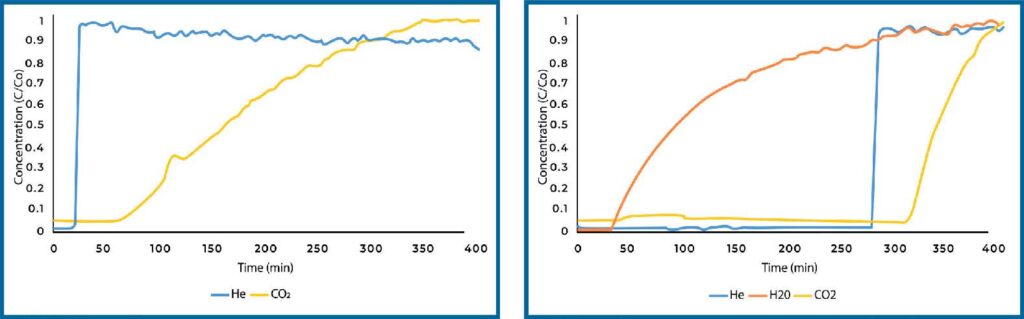
TEPA loaded Si-Al displayed an increase in CO2 adsorption in dry conditions over plain Si-Al, however it adsorbed less than half as much CO2 when compared to PEI loaded Si-Al (see Figure 4). The TEPA loaded samples excels, however, in the presence of humidity, adsorbing more CO2 than any of the other SiAl samples (including those in dry conditions). TEPA is a secondary amine which creates a synergistic effect between the adsorption of CO2 and water enhancing the overall CO2 adsorption capacity.
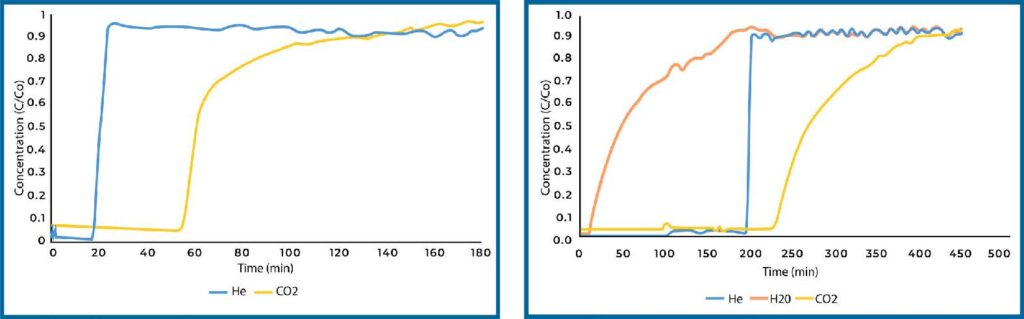
Plain zeolite 13X was also analyzed for CO2 adsorption capacity in both dry and humid conditions (see Figure 5). Zeolite 13X is a microporous material and displayed significantly increased capacity compared to Si-Al in dry conditions. When exposed to humidity, zeolite 13X will preferentially adsorb water over CO2 such that it loses all capacity in the presence of water vapor.
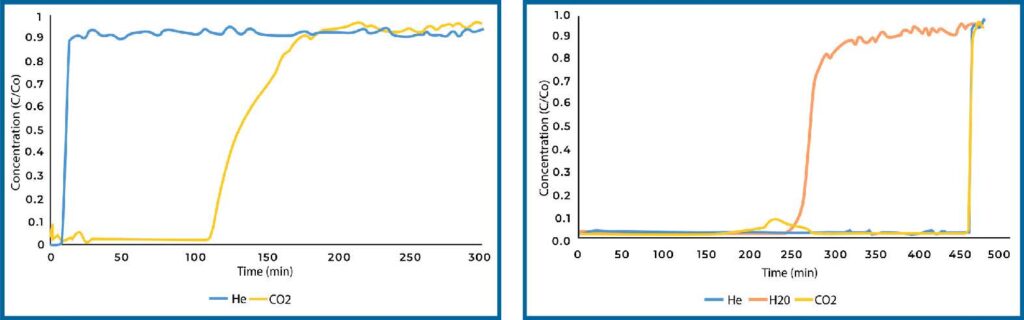
Zeolite 13X loaded with PEI displayed decreased CO2 adsorption in dry conditions compared to the plain sample (see Figure 6). This can be explained by the pore size of zeolite 13X. Zeolite 13X has a much smaller pore size compared to SiAl, as such, loading PEI into the pore space is more difficult and PEI will completely fill the pore space. We see this in the surface area of the materials, where the surface area of zeolite 13X was essentially zero after loading with PEI. In humid conditions, the CO2 adsorption decreased compared to plain zeolite 13X in dry conditions, however it still resulted in and adsorption of 0.19 mmol/g which is significantly more than plain zeolite 13X in humid conditions.
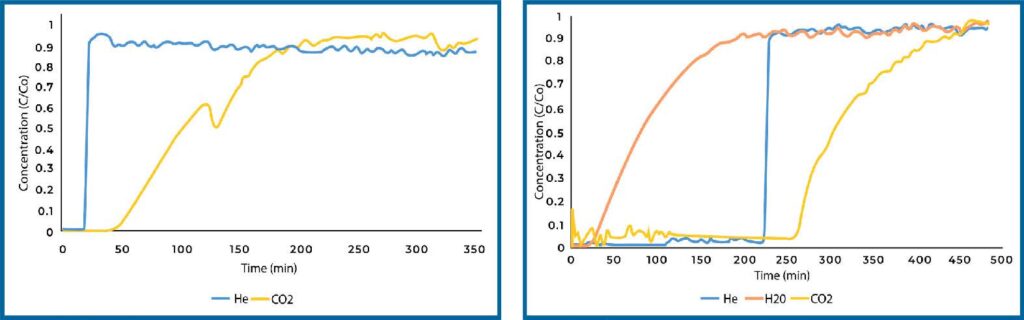
Zeolite 13X loaded with TEPA displayed weaker CO2 adsorption under dry conditions (see Figure 7). This loss in capacity is likely due to TEPA coating the surface of the zeolite and blocking some of the pore space. Under humid conditions, the CO2 adsorption was substantially increased and resulted in the highest capacity among all materials and conditions that were tested, reaching a capacity of 0.31 mm/g.
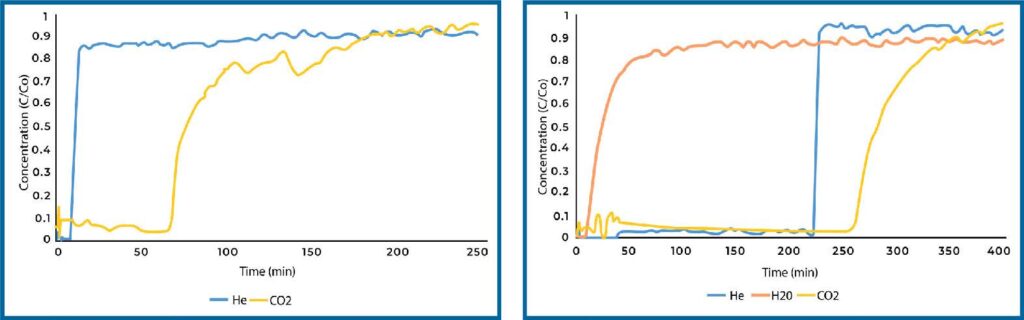
Conclusions
SiAl and zeolite 13X were evaluated for direct air capture in both humid (40% relative humidity) and dry conditions. Both SiAl and zeolite 13X adsorb CO2 at 400 ppm in dry conditions, however their adsorption capacity drops significantly in humid conditions due to competitive adsorption between CO2 and water. PEI and TEPA were used as amine adsorbents for CO2 to improve their adsorption capacity in humid conditions.
SiAl loaded with PEI substantially increased adsorption in both humid and dry conditions. In dry conditions, the CO2 adsorption capacity increased from 0.047 to 0.166 mmol/g. In humid conditions, the capacity increased from 0.028 to 0.058 mmol/g. SiAl loaded with TEPA increased adsorption in dry conditions, but not as significantly as SiAl loaded with PEI. In humid conditons, however, the adsorption capacity increased significantly from 0.028 to 0.198 mmol/g.
Loading zeolite 13X with PEI and TEPA decreased its adsorption capacity for CO2 in dry conditions. Likely this is due to PEI and TEPA coating and filling the pore space of zeolite 13X due to the large size of the amine compared to the small pore size of zeolite 13X. This can also be seen in the decrease in surface area of PEI and TEPA loaded zeolite 13X. Zeolite 13X has strong affinity for water adsorbing water and adsorbed no CO2 in humid conditions. The loading with PEI and TEPA enhanced adsorption under humid conditions reaching a capacity of 0.19 mmol/g and 0.31 mmol/g respectively.
In this study, we can see the advantages that amine loading can provide with respect to direct air capture in humid conditions. While we don’t produce materials for direct air capture at Micromeritics, this app note provides groundwork for collecting breakthrough measurements on a breakthrough system. Table 2 below displays all the analysis that were performed for breakthrough adsorption capacity and the quantity of CO2 adsorbed for each analysis.
Material | Treatment | Test | Quantity Adsorbed (mmol/g) |
---|---|---|---|
SiAl | Plain | Dry | 0.047 |
PEl | Dry | 0.166 | |
TEPA | Dry | 0.072 | |
Plain | 40%RH | 0.028 | |
PEl | 40%RH | 0.058 | |
TEPA | 40%RH | 0.196 | |
Zeolite 13X | Plain | Dry | 0.261 |
PEl | Dry | 0.097 | |
TEPA | Dry | 0.170 | |
Plain | 40%RH | < 0.001 | |
PEl | 40%RH | 0.192 | |
TEPA | 40%RH | 0.314 |