The hybrid nature of metal-organic frameworks provides an almost infinite set of combinations between metal clusters and organic linkers, rendering these porous materials endless possible applications, such as methane storage1, carbon dioxide capture2, hydrogen storage3, and gas separation4. Water adsorption5 in metal-organic frameworks (MOFs) has attracted more attentions in the past decade due to the potential applications of MOFs in air dehumidification6, water capture at low humidity7, and water storage8. With more and more kinetically and thermodynamically water-stable MOFs9,10 being designed and synthesized, the need for material characterization by water vapor adsorption instruments becomes crucial.
Micromeritics 3Flex gas sorption analyzer, recognized as the most advanced instrument in the field of material characterization by gas adsorption, is widely used in research universities, government laboratories, and research & development facilities in the private sector. In addition to the inert gas (nitrogen, argon and krypton) physisorption, static chemisorption, and dynamic chemisorption (TCD or mass spectrometer as detectors); vapor sorption is another widely used and well-trusted option on the 3Flex gas sorption analyzer.
Vapor sorption analysis has the advantages of: 1) faster experiment: experiments take hours or days rather than weeks on gravimetric sorption analyzers; 2) higher throughput: 3Flex with up to three stations can analyze three samples simultaneously even with different pressure tables; 3) easier sample-handling: for moisture-sensitive materials, the transfer of sample from bottle to sample tube can be simply performed with the use of seal frits in a glovebox. The sample will not be exposed to air at all which is difficult to achieve on gravimetric sorption analyzers.
Here we present water vapor adsorption isotherms acquired by Micromeritics 3Flex gas sorption analyzer for two representative MOFs, HKUST-1(Cu-BTC)11 and MIL-1019 . HKUST-1, Cu3 [C6H3(COO)3]2 , is composed of copper (II) paddlewheel dimers linked by trimesic acid trianions and is commercially available. MIL-101, Cr3 XO[C6H4(COO)2]3 (X = F, OH), has trinuclear chromium(III) metal clusters and terephthalic acid dianions. These two MOFs were selected because both HKUST-1 and MIL-101 have coordinatively unsaturated metal sites providing high affinity to water molecules while maintaining their structures intact.
The two water vapor sorption experiments were conducted simultaneously on the same 3Flex instrument with different pressure table setups (P/Po = 0.001-0.90) at 298K. HKUST-1 material was provided by scientists at NuMat Technology. MIL-101 was received as a gift. The material crystallinity was confirmed by the providers. SEM images were acquired at the Particle Testing Authority with a Phenom ProX desktop SEM (Figure 5 and 6). Vacuum degassing was conducted at 170°C overnight. The BET surface areas of HKUST-1 and MIL101 are 1574 m2/g and 1379 m2/g respectively. The steep sorption at low P/Po region and plateau afterward on the nitrogen gas sorption isotherm in Figure 1 demonstrates the microporosity of HKUST-1. The log plot of the nitrogen isotherm of HKUST-1 in Figure 3 exhibits step characteristics which show the interaction between HKUST-1 and gas molecules with strong quadrupole.12,13 Whereas, the nitrogen gas sorption isotherm in Figure 2 indicates the two types of mesopores with internal diameters close to 2.9 and 3.4 nm in MIL-1019 .
With accurate dosing of 10 cm3/g STP on 3Flex, the adsorption on coordinately unsaturated metal sites and following micropore fillings of HKUST-1 are well presented on the water vapor sorption isotherm (P/Po < 0.3) in Figure 1. HKUST-1 has a water capacity of 512 cm3 /g STP (41 wt.%) at P/Po = 0.3, 298K, suggesting water capture as a potential application in low relative humidity environments. The water capacity of HKUST-1 is 648 cm3 /g STP (52 wt.%) at P/Po = 0.90, 298K, exceeding that of conventional water adsorbents such as alumina and zeolite.
MIL-101, on the other hand, attributes most of its water capacity to the higher relative humidity region, P/Po > 0.35, which agrees with its mesoporous nature. MIL-101 has a water capacity of 96.2 cm3 /g STP (7.7 wt. %) at P/Po = 0.3 and a water capacity of 850.5 cm3 /g STP (68.3 wt. %) at P/Po = 0.90. Even though MIL-101 may not be suitable for water capture applications in low humidity environments, it may be utilized for dehumidification in static conditions, as in desiccant packs. The hysteresis is due to the pore fillings occurred through capillary condensation. The large difference in water adsorption quantity of 630 cm3 /g STP (50.6 wt. %) within a narrow range of relative humidity from P/Po = 0.35 to 0.5 reveals potential applications for adsorption heat-pump or chiller14. With a higher pressure and temperature, the hysteresis can be eliminated creating an even narrower range of relative humidity rendering it more suitable for the above mentioned applications.
In addition to the typical water vapor adsorption and desorption isotherms, Micromeritics 3Flex with vapor option are equipped to perform sorbent regenerability and cycling study, heat of adsorption study, and many more with an extensive library of fluid properties of commonly used vapors.
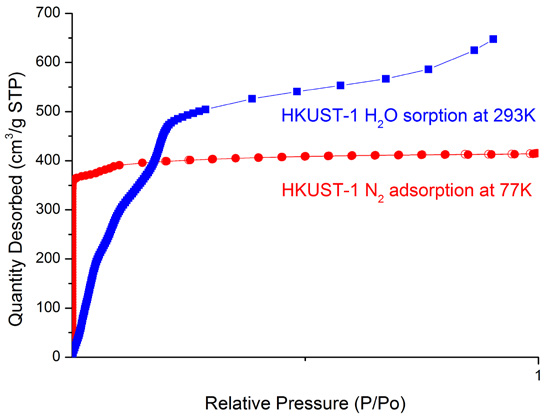
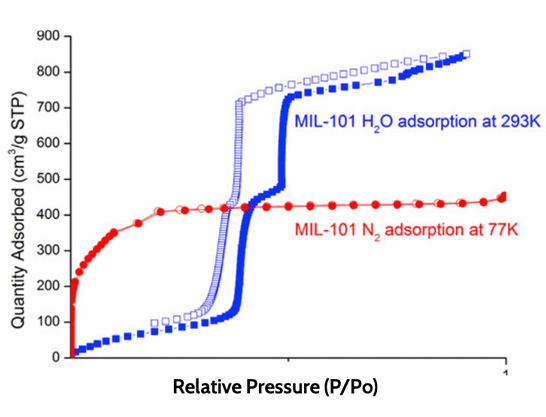
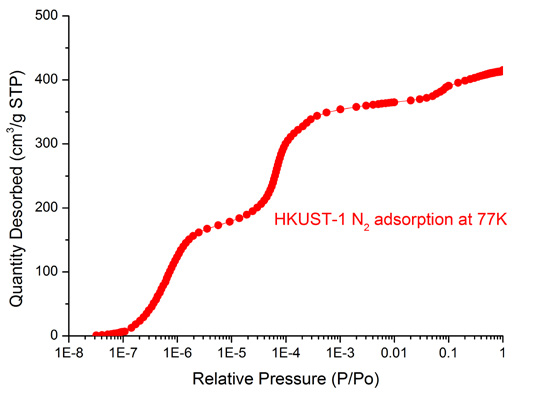
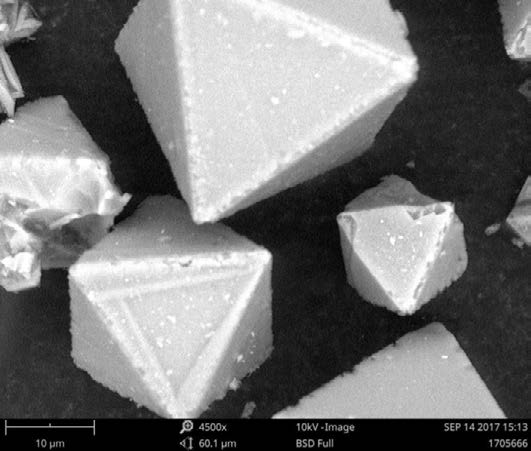
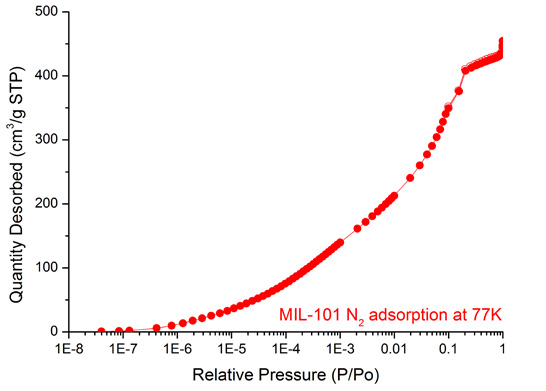
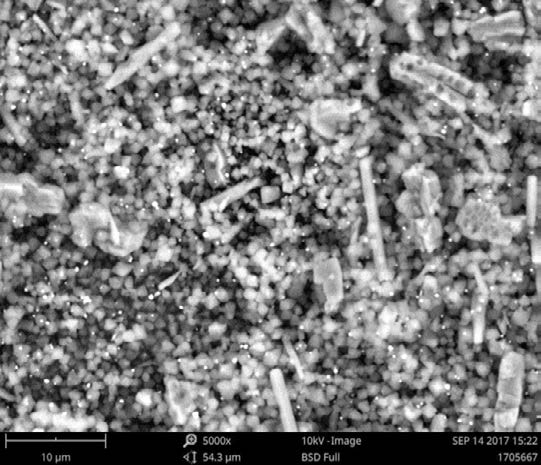
References
(1) Wu, H.; Zhou, W.; Yildirim, T. High-Capacity Methane Storage in Metal−Organic Frameworks M2 (Dhtp): The Important Role of Open Metal Sites. J. Am. Chem. Soc. 2009, 131 (13), 4995–5000.
(2) Mason, J. A.; Sumida, K.; Herm, Z. R.; Krishna, R.; Long, J. R. Evaluating Metal–organic Frameworks for PostCombustion Carbon Dioxide Capture via Temperature Swing Adsorption. Energy Environ. Sci. 2011, 4 (8), 3030–3040.
(3) Collins, D. J.; Zhou, H.-C. Hydrogen Storage in Metal–organic Frameworks. J. Mater. Chem. 2007, 17 (30), 3154–3160.
(4) Adams, R.; Carson, C.; Ward, J.; Tannenbaum, R.; Koros, W. Metal Organic Framework Mixed Matrix Membranes for Gas Separations. Microporous Mesoporous Mater.2010, 131 (1), 13–20.
(5) Küsgens, P.; Rose, M.; Senkovska, I.; Fröde, H.; Henschel, A.; Siegle, S.; Kaskel, S. Characterization of Metal-Organic Frameworks by Water Adsorption. Microporous Mesoporous Mater. 2009, 120n (3), 325–330.
(6) Guo, P.; Wong-Foy, A. G.; Matzger, A. J. Microporous Coordination Polymers as Efficient Sorbents for Air Dehumidification. Langmuir 2014, 30 (8), 1921–1925.
(7) Kim, H.; Yang, S.; Rao, S. R.; Narayanan, S.; Kapustin, E. A.; Furukawa, H.; Umans, A. S.; Yaghi, O. M.; Wang, E. N. Water Harvesting from Air with Metal-Organic Frameworks Powered by Natural Sunlight. Science 2017, eaam8743.
(8) Seo, Y.-K.; Yoon, J. W.; Lee, J. S.; Hwang, Y. K.; Jun, C.-H.; Chang, J.-S.; Wuttke, S.; Bazin, P.; Vimont, A.; Daturi, M.; et al. Energy-Efficient Dehumidification over Hierachically Porous Metal–Organic Frameworks as Advanced Water Adsorbents. Adv. Mater. 2012, 24 (6), 806–810.
(9) Férey, G.; Mellot-Draznieks, C.; Serre, C.; Millange, F.; Dutour, J.; Surblé, S.; Margiolaki, I. A Chromium TerephthalateBased Solid with Unusually Large Pore Volumes and Surface Area. Science 2005, 309 (5743), 2040–2042.
(10) Cavka, J. H.; Jakobsen, S.; Olsbye, U.; Guillou, N.; Lamberti, C.; Bordiga, S.; Lillerud, K. P. A New Zirconium Inorganic Building Brick Forming Metal Organic Frameworks with Exceptional Stability. J. Am. Chem. Soc. 2008, 130 (42), 13850–13851.
(11) Chui, S. S.-Y.; Lo, S. M.-F.; Charmant, J. P. H.; Orpen, A. G.; Williams, I. D. A Chemically Functionalizable Nanoporous Material [Cu3(TMA)2 (H2O)3 ]N. Science 1999, 283 (5405), 1148–1150.
(12) Wu, H.; Simmons, J. M.; Srinivas, G.; Zhou, W.; Yildirim, T. Adsorption Sites and Binding Nature of CO2 in Prototypical Metal−Organic Frameworks: A Combined Neutron Diffraction and First-Principles Study. J. Phys. Chem. Lett. 2010, 1 (13), 1946–1951.
(13) H. Hendon, C.; Walsh, A. Chemical Principles Underpinning the Performance of the Metal–organic Framework HKUST-1. Chem. Sci. 2015, 6 (7), 3674–3683.
(14) Ehrenmann, J.; Henninger, S. K.; Janiak, C. Water Adsorption Characteristics of MIL-101 for Heat-Transformation Applications of MOFs. Eur. J. Inorg. Chem. 2011, 2011 (4), 471–474