Dr. Darren Lapham, MCA Services, Cambridgeshire, UK.
This application note describes the analysis of full gas adsorption / desorption isotherms of carbon black samples using a Micromeritics 3Flex micropore instrument. From the isotherms, BET surface area together with micro-pore, meso-pore and small macro-pore size distributions can be ascertained along with pore volume and pore area. The use of carbons is commonplace throughout many industries and applications. Carbons, often as carbon blacks, play an essential role in a range of energy production and storage devices such as re-chargeable batteries, fuel cells and super-capacitors. They serve as electro-catalysts, catalyst supports and membrane materials. They may be present as pure carbon or doped / impregnated with various metal oxides or precious metals. The use of carbon in the form of activated carbon, carbon black, carbon nano-tubes, graphene, graphite etc, are all being explored or utilised at present. Optimisation of electrode porosity is essential as it directly affects electrolyte transport within the electrodes, the number and availability of active electrode sites, electrode conductivity, and the intercalation of charge carrier species. Cyclability can also be maximized through minimising pore blocking BET surface area and total pore volume are very common measurements which feature in the characterisation of anode and cathode materials. However, the significance of the porous characteristics of both raw materials, intermediates and finished electrodes extends beyond these: the pore size distribution and pore area must also be considered and will provide a more complete understanding of the porous nature of the materials. Three commercially available carbon black powders known for their conductive properties and targeted at battery applications have been analysed. Nitrogen adsorption and desorption isotherms were measured using a microporeequipped Micromeritics 3Flex instrument. Samples were first degassed using a Micromeritics VacPrep at 300°C for 6 hours at an ultimate vacuum < 100 mTorr before transferring to the 3Flex and then continued under vacuum at 300°C for a further 16 hours. Analyses were undertaken at 77K, with isothermal jackets being used for each sample tube, freespace was measured with He at the end of analysis. Adsorption isotherms were measured by a combination of dosing methods. The fixed dosing option was used at 1 cc/g STP up to 0.005 P/Po at 45 s equilibration interval and then 0.5 cc/g STP up to 0.01 P/Po at 30 s equilibration interval. The remainder of the adsorption isotherm to 0.995 P/Po and subsequent desorption measurement to 0.05 P/ Po were undertaken using the incremental dosing method. Samples were weighed after analysis and this mass applied retrospectively to the analysis files before report generation. Figure 1 shows the linear isotherm plots and figure 2 shows the logarithmic isotherm plots. The logarithmic plots are particularly useful for quickly ascertaining differences in adsorption within the micro-pore region of the isotherms.
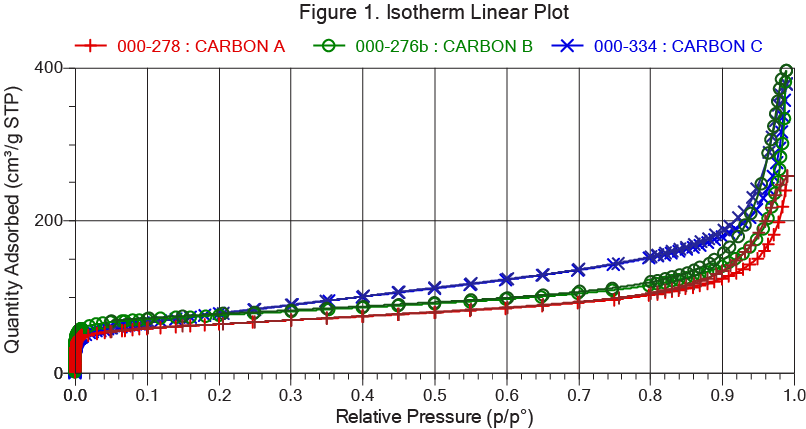
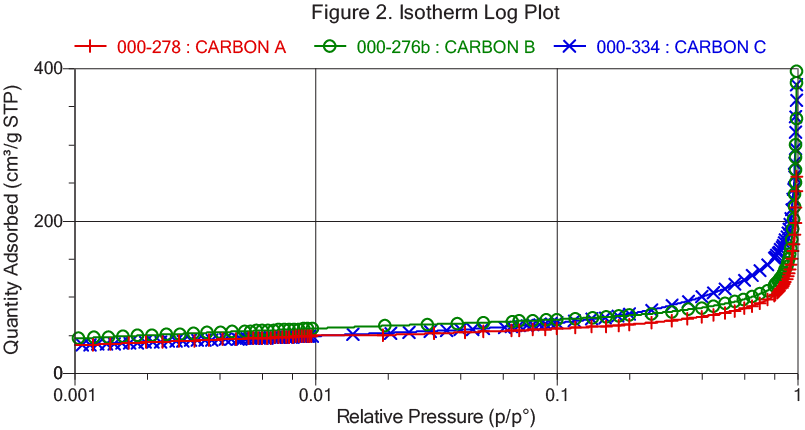
Micromeritics Microactive software was used to select the most appropriate methods and models for determining pore area, volume and size data. Important data for characterising the porous nature of the materials are summarised in the table below, the samples being labelled A to C in order of increasing BET surface area.
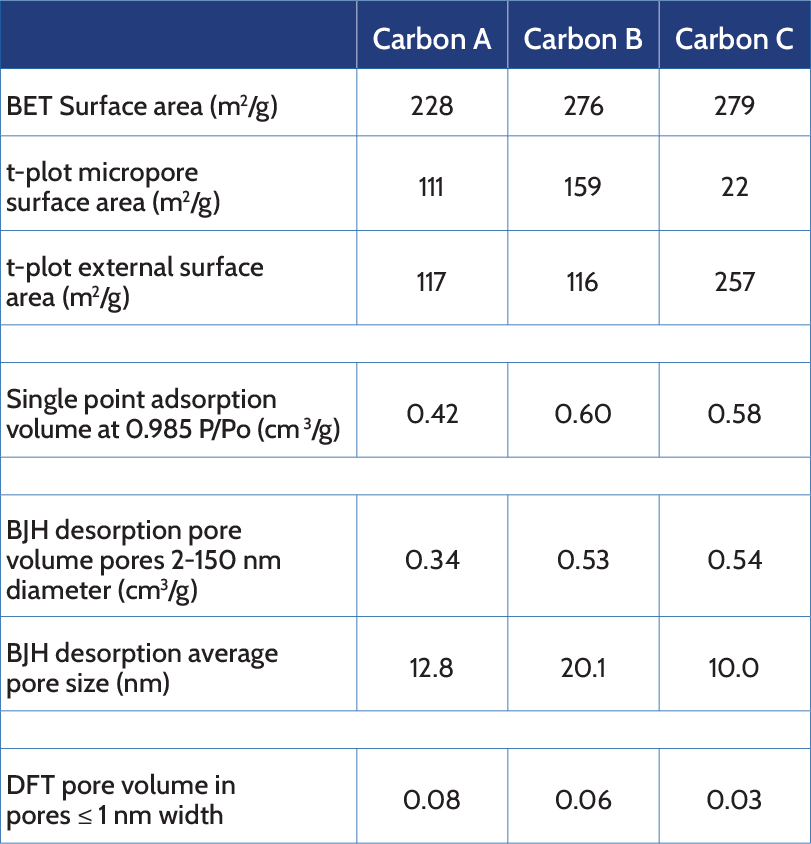
BET surface area is universally reported when analysing carbon materials. Further to this, de Boer t-plots have also been constructed which enable the contribution of micropore area to total surface area to be calculated. 2D DFT models were applied to account for variation in the aspect ratio of carbon pore geometries. Pore size, volume and area data were reported by a combination of methods: DFT was applied to the Micropore (< 2nm diameter) range, the pore size distribution is shown in Figure 3. BJH, shown in Figure 4, was applied to mesopore (2-50 nm diameter) and small macropore (50 – 150 nm diameter) ranges.
The BET surface area of carbons B and C are virtually identical whilst that of carbon A is 17% lower. However, the pore size and volume distributions, the extent of micro-porosity and therefore the source of surface area are profoundly different.
Considering surface area, the t-plot data are particularly useful: Carbon C has the lowest contribution of micropore area to overall surface area, approximately 8% of total surface area being located within micro-pores. Carbons A and B on the other hand have a more equal contribution of micropore area to total surface area, 49% and 58% respectively.
Pore size is critical to many applications of carbons as it often directly affects performance and, in addition to pore area, can be considered through the distribution of pore sizes and pore volume. Micro-pores can be investigated using the DFT method and this shows the pore size distributions to be similar. However, significantly, carbon A has the highest micropore volume with the majority of micro-pores located in particularly small pores (< 0.7nm width). Carbon C on the other hand has the lowest micropore volume with pores present within two size ranges: < 0.7nm and > 0.7nm width with similar volumes in each. Carbon B can be considered as being intermediate with respect to both micropore size and volume.
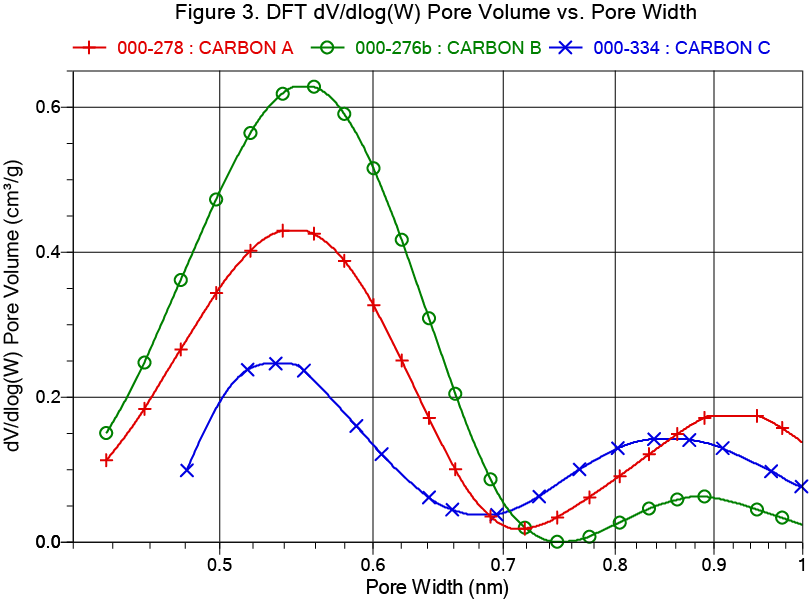
The extent of meso-porosity and macro-porosity must also be considered, especially since these pores are often present as ‘transport pores’, providing access to any micro-pores within a porous network. BJH pore models are particularly useful in this respect. All three carbons have appreciable porosity in the range 2 – 150 nm diameter, although the pore volume for carbon A is considerably lower than for carbons B and C. Whilst the volume of pores is very similar for carbons B and C, the average pore size present in carbon B is double that of carbon C.
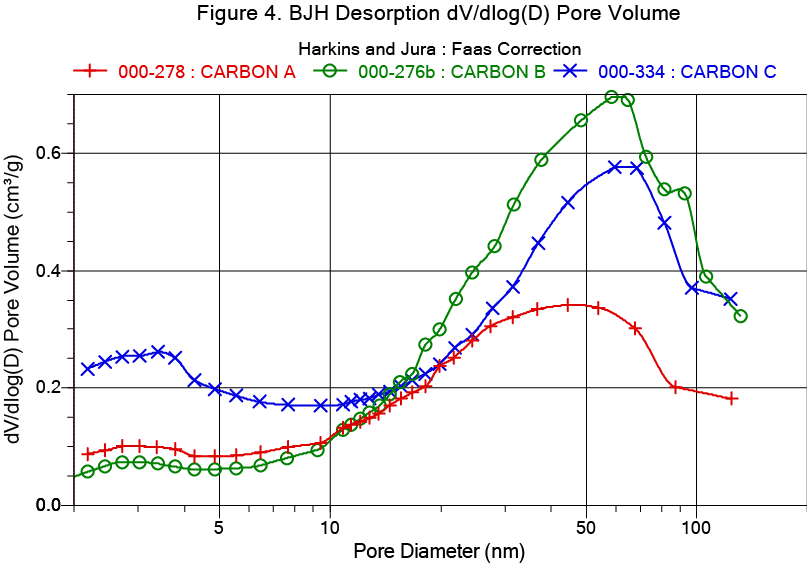
Despite the similarity in BET surface area, there are some very significant differences in pore sizes and volumes which are likely to have profound effects on the performance of the carbons. The separation of micro-pore and meso / macro-pore data is important. The higher micro-porosity of Carbons A and B may provide for shortened diffusion pathways through the material and thus faster electron transfer with improved conductivity as well as an abundance of active sites for electrochemical processes. Conversely, higher micro-pore volume located within particularly small pores, as with Carbon B, may make the material more prone to pore blocking and clogging during use and thus reduce lifetime. The relative absence of micro-porosity within Carbon C indicates that this material would be least prone to pore blocking and clogging. Meso and macro-porosity often provide essential pathways to micro-pores: Carbon B could, therefore, be expected to show improved transport of charge carrier species, for example Li+, to and from active sites providing that the smallest micro-pores do not themselves limit diffusion. Carbon C could be expected to show good charge carrier transport properties due to appreciable meso-porosity but may have a lower concentration of active sites due to the relative lack of micro-porosity. It is likely that selection of the best carbon will be dependent on the precise application. For example, Carbon A may be more suited to Li-Ion battery cathodes where a balance of micro and meso-porosity is demanded with control over micro-pore size. It would also be a good selection where doping with, for example, a metallic species is required. Carbon C appears to be particularly suited to anode fabrication throughout a range of device types, where high surface area will provide for fast charge carrier transfer. Carbon B may be applicable to either anode or cathode fabrication but effects of the particularly small micro-pores would require further investigation of system performance. Knowledge of key differences in porosity is essential to the understanding of the selection, application and performance of carbon materials. This can only be realised when the entire isotherm is collected and considered through the application of a variety of pore models.
About Dr. Lapham:
Dr. Darren Lapham Ph.D has been Operations Manager at MCA Services, a UK contract analysis laboratory and consultancy for physical materials characterization since 2002. Darren specializes in techniques of gas adsorption, mercury porosimetry and chemisorption. Darren obtained his Ph.D in Physical Chemistry from the University of Essex in 2000 and has undertaken post-doctoral research at Greenwich University and the University of Cambridge, working on semi-conducting and solid state gas sensors and rechargeable battery technology. Currently, Darren is researching the physical characterization of pharmaceuticals and battery components.