Ambalavanan Jayaraman and Ralph T. Yang*
*Department of Chemical Engineering, University of Michigan Ann Arbor, Michigan 48109, USA
Introduction
Upgrading production from the aging natural gas wells by non-cryogenic separation of nitrogen from methane continues to be a topic of intense research. New nano-structured sorbent materials have been developed in the recent past for the purpose of separation of nitrogen/methane mixtures by pressure swing adsorption (PSA). Ackley and Yang have demonstrated the use of carbon molecular sieve (CMS) for this purpose but have also shown that the potential for CMS to achieve the pipeline quality is doubtful [1]. Habgood developed a process using 4A molecular sieves for the same separation, but this process was limited to low temperatures (273 K) and high methane content [2]. Although separation can often be improved through process optimization, maximum performance is limited by the adsorption characteristics of the sorbent. More promising separation of N2/CH4 mixtures was achieved by PSA using calcium-exchanged clinoptilolite, which is a naturally occurring zeolite [3]. The Molecular Gate Technology is the most promising process available commercially at present for N2/CH4 separation and it uses Sr-ETS-4 calcined at 315 oC [4].
Clinoptilolites have a two-dimensional channel structure with multiple indigenous cations located in these channels that can be systematically altered to get desired adsorption characteristics by performing ion exchange [5]. One of the objectives of this work is to prepare mixed ion-exchanged clinoptilolites (i.e., partially exchanged clinoptilolites) and test their effectiveness for CH4 enrichment by means of adsorption measurements. In this study various ion-exchanged forms of clinoptilolites are prepared and the low-pressure adsorption kinetics of nitrogen and methane in them is studied using Micromeritics’ ASAP 2010 (constant volume apparatus).
Experimental
Clinoptilolite (TSM 140 D clinoptilolite) supplied by Steelhead Specialty Minerals (Spokane, WA) was used. The raw clinoptilolite was crushed to 140 mesh, purified and then subjected to ion exchange with various salt solutions following procedures described elsewhere [6]. Partially exchanged and mixed ion exchange clinoptilolites were prepared by sequential ion exchange, complete details given elsewhere [6,7].
The rate of adsorption of nitrogen and methane on the various clinoptilolite samples prepared were measured using Micromeritics’ ASAP 2010 system, equipped with the Rate of Adsorption (ROA) software. Prior to measurements, the clinoptilolites were dehydrated in vacuum at 350 oC. All diffusion data were measured at a stepped pressure increment, from 0 to 0.05 atm. For each pressure increment the ROA software reports the amount adsorbed as a function of time. From this the fractional uptake is calculated as follows:
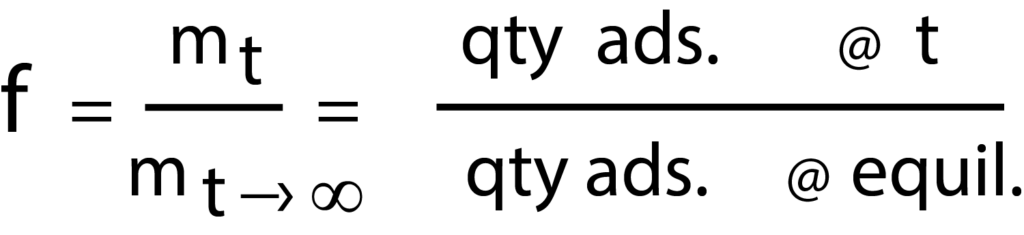
Whereas, fractional uptake (f) equals the quantity adsorbed at time divided by the quantity adsorbed at equilibrium.
The initial few data points were not used in the analysis since there are interfering dynamics in them; namely, system dynamics – due to the rapid expansion of the gases when dosing the sample chamber; and sensor dynamics – due to the transducer diaphragm being subjected to rapid pressure change. Calibration of these interferences was carried out by doing blank adsorption runs on the Micromeritics ASAP 2010 with aluminum foil and the dynamics were found to last for a maximum of 4 seconds. Hence the Micromeritics ASAP 2010 could be used with confidence for ROA measurements where the time scale of adsorption differs from that of the interfering dynamics by orders of magnitude. Diffusion time constants were then calculated by fitting experimental fractional uptake data with the solution of the diffusion equation for spherical particles [8].
Results and Discussion
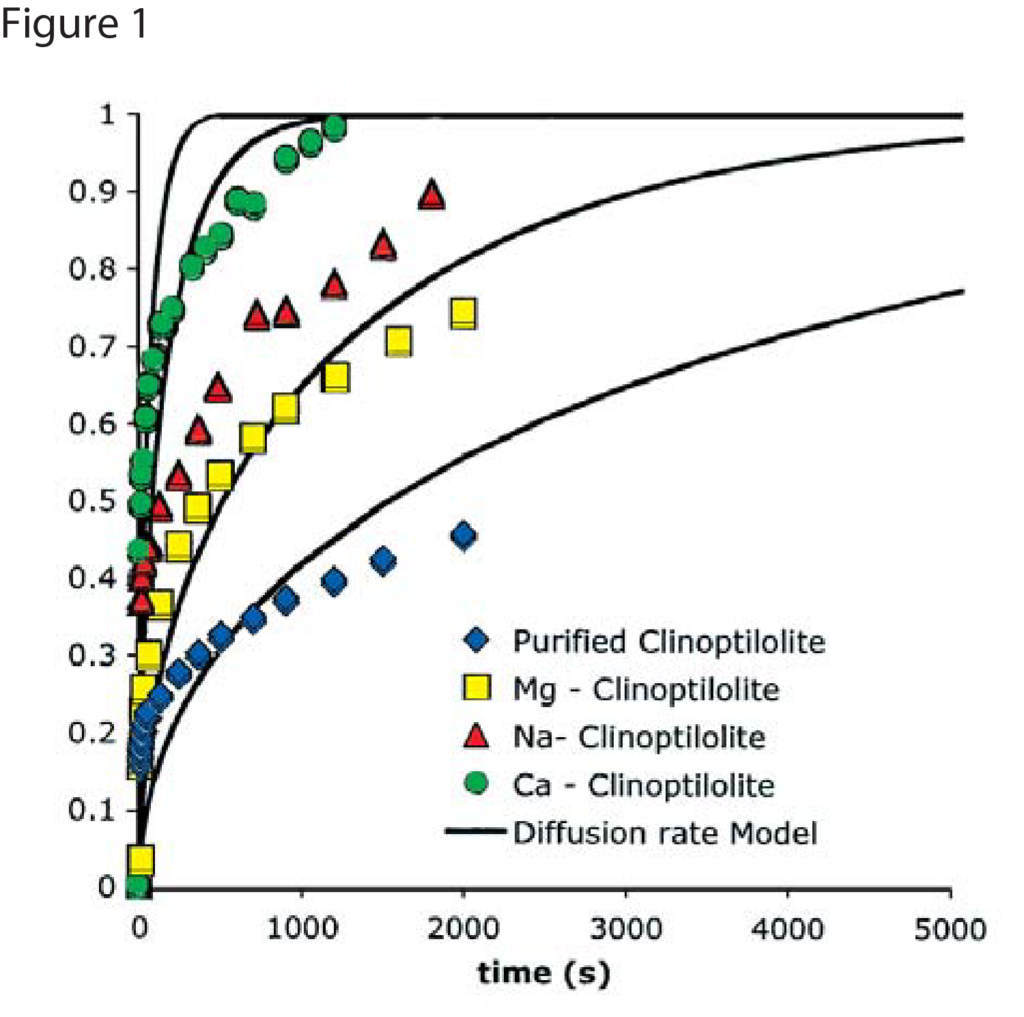
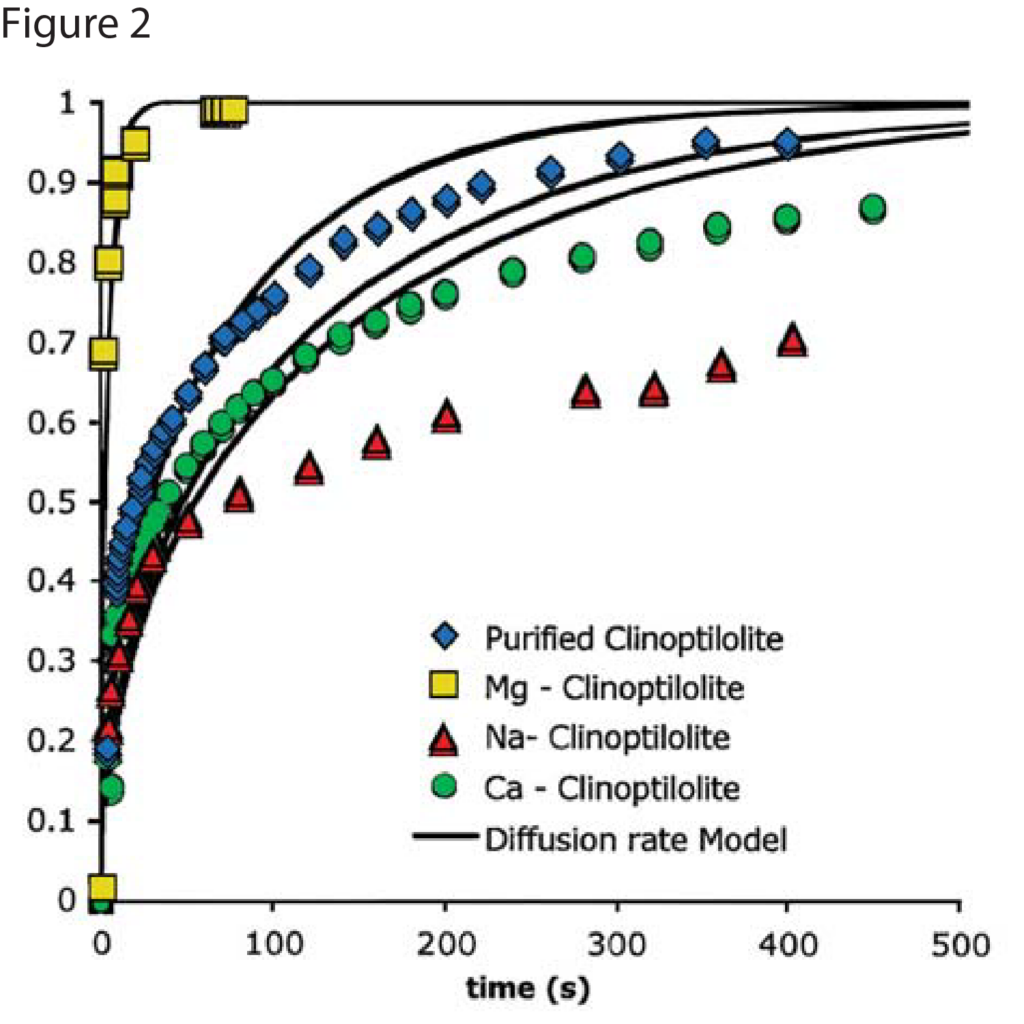
Figures 1 and 2 show nitrogen and methane uptake curves on some of the clinoptilolite samples, respectively. The diffusional time constants for nitrogen and methane uptake along with their kinetic selectivity on various clinoptilolite samples are given in Table 1. We could see that nitrogen diffuses faster than methane in most of the clinoptilolites and the diffusional time constants differ by orders of magnitude depending on the exchange cation. Clinoptilolite framework consists of two parallel channels of ten-member (Channel A) and eight-member (Channel B) rings that are connected to a third eight-member ring (Channel C). The cations compete for cation exchange sites that are located in these three channels. In both Ca-exchanged clinoptilolite and Na-exchanged clinoptilolite, the nitrogen diffusion is slower by a couple of orders of magnitude compared to other clinoptilolites implying severe pore blocking in the channels occupied by the Ca2+ and Na+ cations (Channels A and B). The fit of the rate model for nitrogen uptake is poor in Na – Clinoptilolite due to the severe pore blocking in channels A and B (explained earlier) while channel C is open which gives rise to rapid initial uptake of both nitrogen and methane. In K – exchanged clinoptilolite samples, both nitrogen and methane diffuse faster since K+ cations occupy site M(3) that, in turn, open up the ten-member ring (Channel A). Mg – exchanged clinoptilolite shows the maximum kinetic selectivity among the clinoptilolite samples studied here, since Mg2+ cations occupy site M(4) located in Channel A, which would ensure molecular sieving of methane in the ten-member ring channel. The adsorption measurements in Micromeritics’ ASAP 2010 aid in understanding the cations, their exchange sites, and their influence on adsorption and diffusion characteristics in clinoptilolite samples, which can be used to tailor the clinoptilolite samples for specific separation needs.
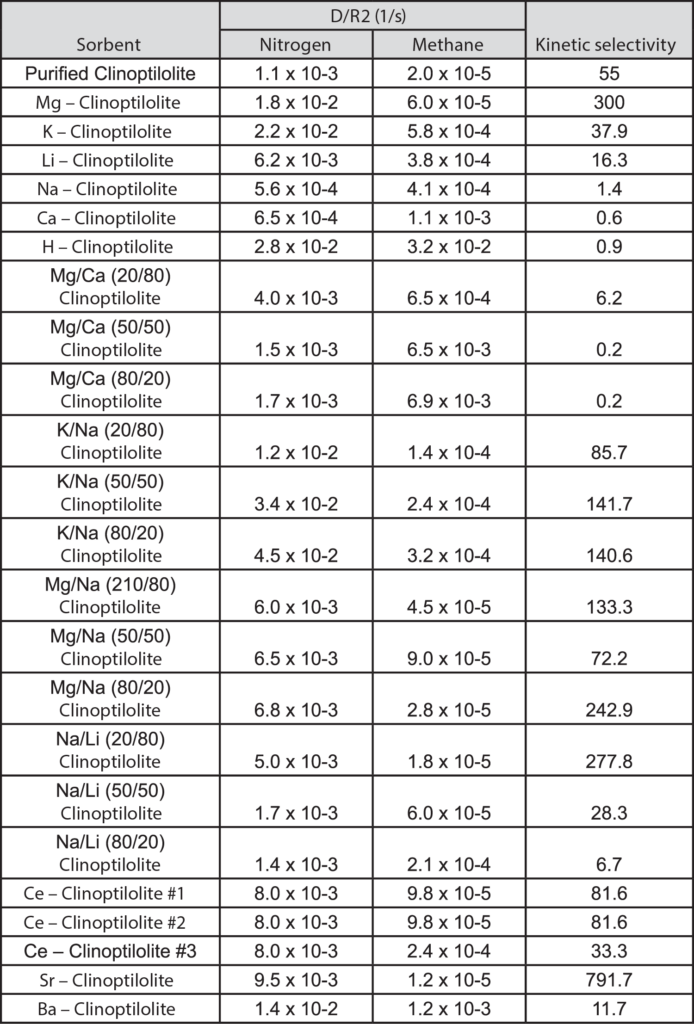
References
[1] Ackley, M. W.; Yang, R. T. AIChE J. 36 (1990), 1229-1238.
[2] Habgood, H. W. Can. J. Chem. 36 (1958), 1384-1397.
[3] Frankiewicz, T. C.; Donnelly, R. G. Industrial Gas Separations. Vol. 1, p. 213 Whyte, T. E., Jr., et al. (Eds.), American Chemical Society: Washington, DC (1983)
[4] Kuznicki, S. M.; Bell, V. A.; Nair, S.; Hillhouse, H. W.; Jacubinas, R. M.; Braunbarth, C. M.; Toby, B. H.; Tsapatsis, M. Nature. 412 (2001), 720-724.
[5] Ackley, M. W.; Yang, R. T. AIChE J. 37 (1991), 1645-1656.
[6] Jayaraman, A.; Hernandez- Maldonado, A. J.; Yang, R. T.; Chinn, D.; Munson, C. L.; Mohr, D. H. Chem. Eng. Sci. 59 (2004), 2407-2417.
[7] Jayaraman, A; Yang, R. T., Ind. Eng. Chem. Res. 44 (2005) 5184-5192.
[8] Do, D. D. Adsorption Analysis: Equilibria and Kinetics, Imperial College Press, London (1998).