Temperature-programmed reduction (TPR) is a widely used tool for the characterization of metal oxides, mixed metal oxides, and metal oxides dispersed on a support. The TPR method yields quantitative information of the reducibility of the oxide’s surface, as well as the heterogeneity of the reducible surface. TPR is a method in which a reducing gas mixture (typically 3% to 17% hydrogen diluted in argon or nitrogen) flows over the sample. A thermal conductivity detector (TCD) is used to measure changes in the thermal conductivity of the gas stream. The TCD signal is then converted to concentration of active gas using a level calibration. Integrating the area under the concentration vs. time (or temperature) yields total gas consumed.
Figure 1 shows a TPR profile for the reaction; MxOy + yH2 → xM + yH2O where MxOy is a metal oxide. This figure illustrates a TPR spectrum where the peak maximum indicates the temperature that corresponds to the maximum rate of reduction. The TPR method provides a qualitative, and sometimes quantitative, picture of the reproducibility of the catalyst surface, as well as its high sensitivity to chemical changes resulting from promoters or metal/support interactions.
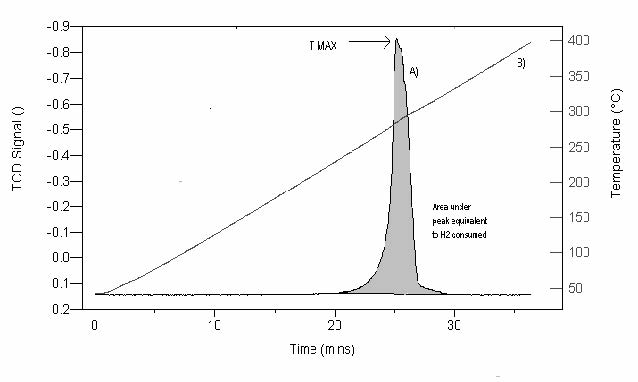
oxide. Trace A displays the TCD signal output as a function of time.
Trace B displays the temperature as a function of time during a 10 °C
heating rate from ambient to 400 °C.
Therefore, the TPR method is also suitable for quality control of different catalyst charges since deviations in manufacturing methods often result in different reduction profiles. Figure 2 illustrates a TPR profile for a reagent grade silver oxide (AgO) sieved to a minus 325 mesh. These data were generated using the AutoChem and show the recorded thermal conductivity signal as a function of the temperature. The specific reaction is AgO + H2 → Ag + H2O. Thirty-six analyses of this particular batch of silver oxide were performed using two different AutoChems. For these 36 analyses, the average Tmax and H2 consumed were:
Average | Sigma | |
Tmax | 119.43 °C | 7.23 |
H2 consumed | 95.39 cc/STP | 1.47 cc/STP |
The theoretical hydrogen consumption for this reaction is 96.72 cc of hydrogen at STP. Thus, the experimental H2 consumption as measured in this series of experiments was 99.7% of theoretical. TPR ultimately yields a bulk reduction of the sample; the peak maxima are an indication of the reducibility of the metal oxide phase. Careful observation of Figure 2 shows a small, broad peak at higher temperatures than Tmax. This peak is attributable to the reduction of some bulk oxide in the sample. The specific particle size of the sample is an important experimental variable; in fact, for bulk oxides an increase in Tmax is predicted for an increasing particle size. TPR results are greatly influenced by 1) the programmed heating rate, 2) H2 concentration in the flowing gas stream, and 3) the flow rate of the gas itself. For example, as the heating is increased, Tmax would also increase. Decreasing the hydrogen concentration in the flowing gas or decreasing the flow rate of the reducing gas would also increase Tmax. Therefore, precise control over these experimental variables, as is available in the AutoChem, is required when attempting to compare data taken in different laboratories.
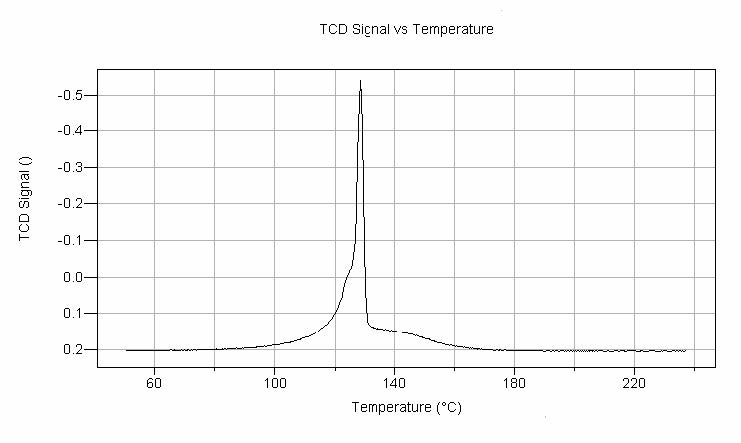
The TCD signal is shown as a function of temperature.
One of the most significant advantages of temperature-programmed methods such as TPR, is that they are very sensitive probes of oxidic surfaces such as supported catalysts. Temperature-programmed methods are some of the best and quickest methods to fingerprint a metal oxide or a supported metal catalyst, and have become extremely valuable and economic methods for catalyst characterization. TPR, in particular, is a very sensitive characterization technique for determining the condition of a catalyst, particularly when using a new catalyst preparation or modifying a catalyst. The structural sensitivity of the TPR method is illustrated in Figure 3. This is the recorded spectrum for the reduction of a binary-mixed metal oxide catalyst of copper and manganese. The profile was obtained with 10% H2 in argon flowing at 50 sccm with a linear heating rate of 10 °C per minute. The four peak areas were obtained by a simple valley-to-baseline integration; the volume of hydrogen consumed was calculated by a TCD concentration previously obtained.
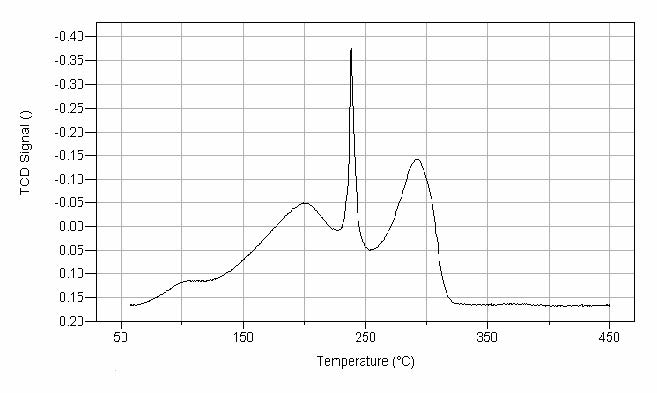
manganese oxide catalyst. The trace displays the TCD signal as a
function of temperature.