Introduction
The use of carbon dioxide allows for rapid, high-resolution characterization of microporous materials. The Micromeritics TriStar II 3020 can be used to characterize micropores below 10 angstroms within 8 hours using CO2 as the adsorptive gas and an ice bath (273 K) for the analysis bath. Isotherms from CO2 adsorption were compared with N2 adsorption isotherms at 77.3 K. Density functional theory (DFT) models for nitrogen and carbon dioxide on slit-pore carbon were used for the pore-size-distribution calculations.
Slow diffusion (equilibration) rates—on the order of hours to days—may be observed during adsorption at cryogenic temperatures on materials with small pores. Strong adsorption potentials in confined pores result in high uptake of adsorptives, such as nitrogen, which corresponds to the filling of micropores at very low pressures that can challenge the lower sensitivity limits of one-torr pressure transducers. Analysis with carbon dioxide addresses both of these issues by having faster equilibration rates, due to increased temperature, and gas uptake at much higher pressures.
Experimental
Two readily available carbon samples, Carboxen® 1012 from Supelco and a sample of MAST synthetic carbon, were used. A Micromeritics TriStar II 3020 was the principle instrument used for CO2 analyses up to 760 torr (relative pressure of 0.03). A Micromeritics ASAP 2050 high-pressure sorption analyzer was used to measure CO2 isotherms up to 7600 torr, and a Micromeritics ASAP 2020 was used to verify micropore sizes using DFT calculations from nitrogen isotherms at 77 K. Bone-dry carbon dioxide, prepurified nitrogen, and ultra-high purity helium were used during the analyses.
Results and Discussion
Figure 1 shows the nitrogen and carbon dioxide isotherms of the Carboxen 1012 and MAST synthetic carbon. Nitrogen adsorption on Carboxen 1012 displays type I isotherm behavior, while the MAST carbon displays type IV isotherm behavior. The trend of enhanced gas uptake by the Carboxen 1012 is observed with the carbon dioxide sorption as well. Mesopores of the MAST carbon and larger micropores, between 10 and 20 angstroms, of the Carboxen 1012 carbon are not shown for clarity.
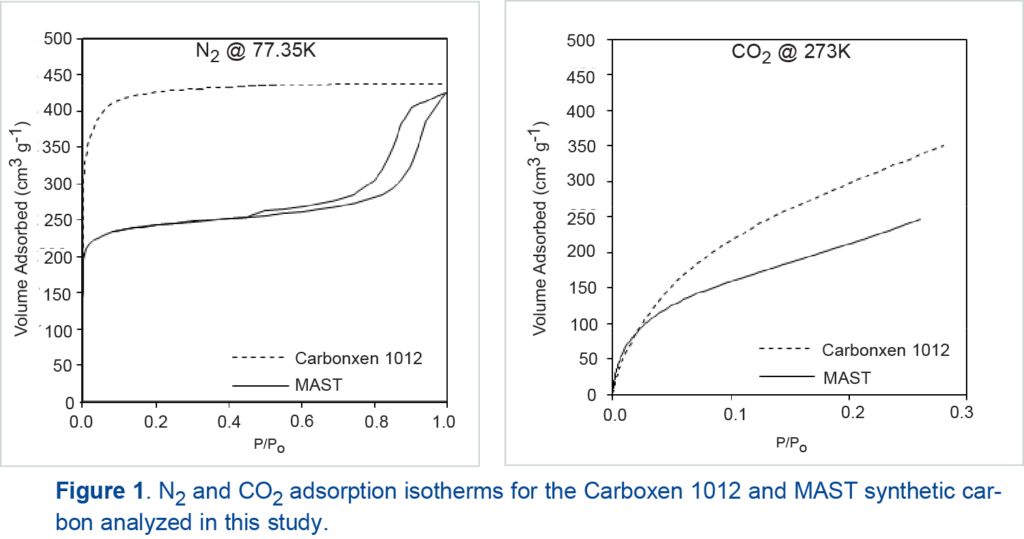
The resolution of the micropore distributions is shown in Figure 2. When compared to nitrogen physisorption, features of the pore size distribution are captured in much greater detail with DFT calculations from carbon dioxide sorption, and pore filling below 7 Å is observed.
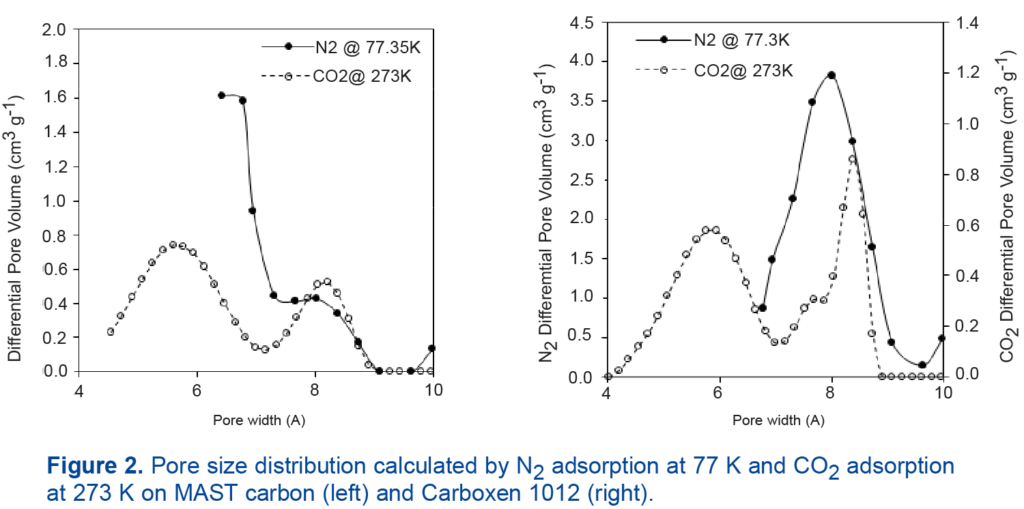
Figure 3 shows the isotherms of carbon dioxide up to 760 torr compared with the measurements up to 7600 torr—demonstrating the agreement of high-pressure and “low-pressure” adsorption.
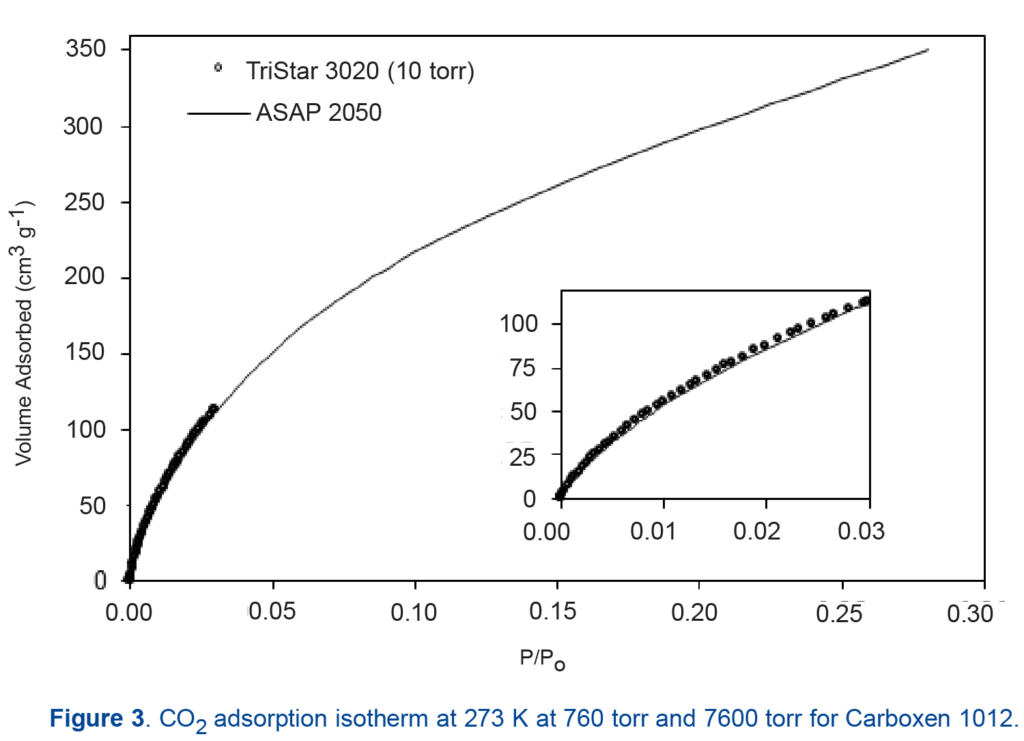
Table 1 shows the surface area calculations from carbon dioxide sorption compared with calculations from nitrogen sorption. BET surface area calculations were performed below 0.1 relative pressure in order to make the results physically meaningful, a common procedure for microporous materials. The Langmuir surface areas from CO2 adsorption were calculated over an absolute pressure range of 600-3000 torr. Langmuir surface areas (not shown) from N2 adsorption, calculated using pressures up to an absolute pressure of 50 torr, agreed within 6% with the nitrogen BET surface areas.
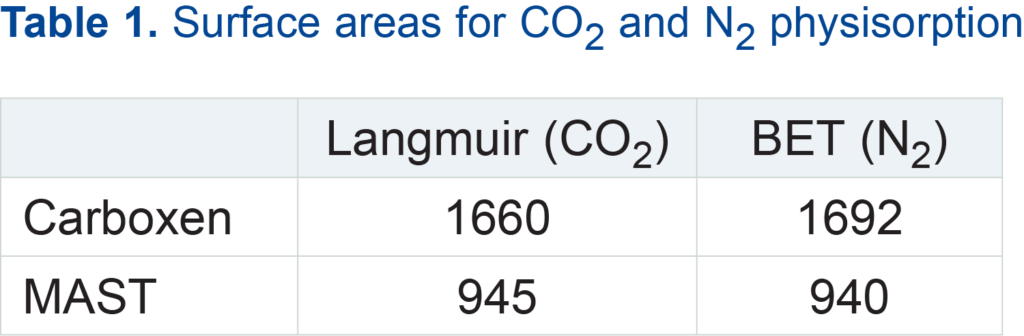
Conclusions
From the data presented, it can be concluded that the TriStar II 3020, as well as other Micromeritics physisorption instruments, can be used to obtain rapid, high-resolution characterization of micropores in carbons. The technique of carbon dioxide physisorption at 273 K can be extended to high pressure, allowing for Langmuir and BET surface areas to be calculated. For the two materials analyzed in this work, the Langmuir surface areas calculated from CO2 adsorption at 273 K are comparable to BET surface areas calculated from N2 adsorption at 77.3 K.